Research
Covalent Organic Frameworks — Growth, Application, and New Science
Covalent organic frameworks (COFs) are crystalline porous polymers with 2D or 3D reticulated networks, recently drawing much attention owing to their unique properties. They particularly excel in design flexibility by which COFs has shown promise in broad applications including energy storage, gas storage/separation, and drug delivery. Our group commenced a few years ago studies of growth optimization, property elucidation, and application development. These projects are currently undergoing and some of them will be presented soon.
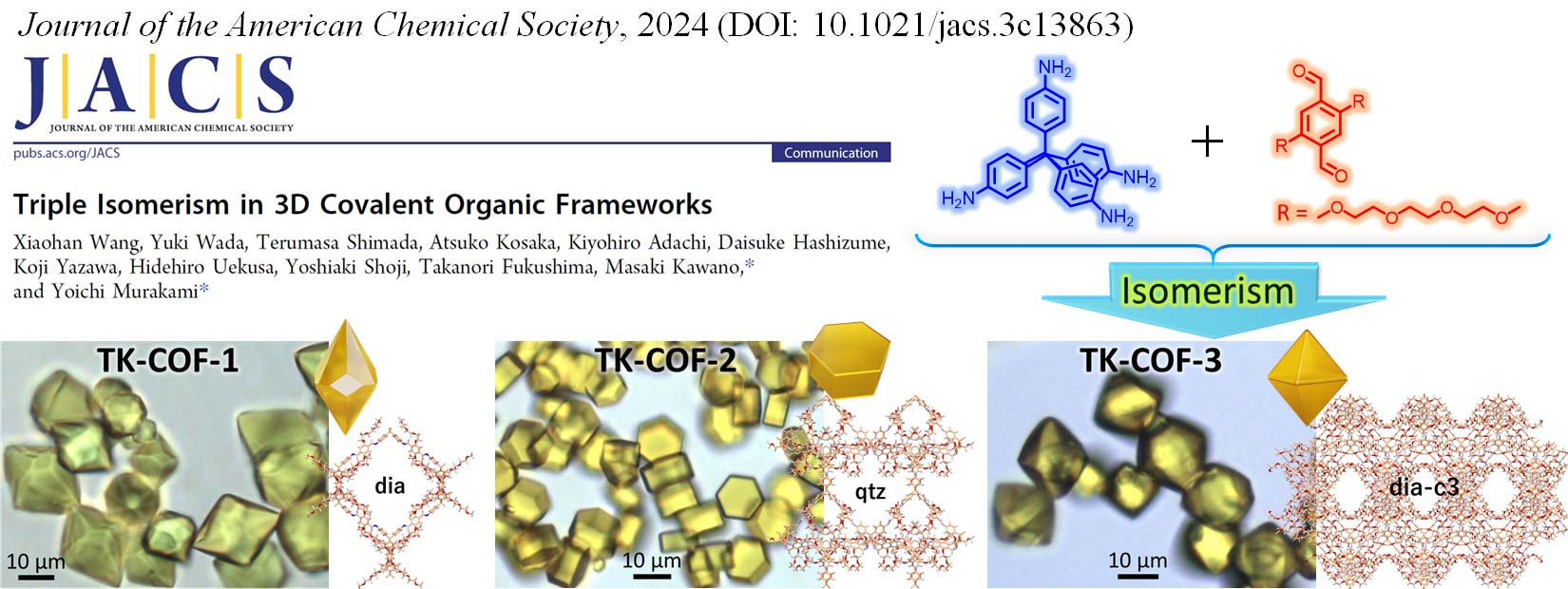
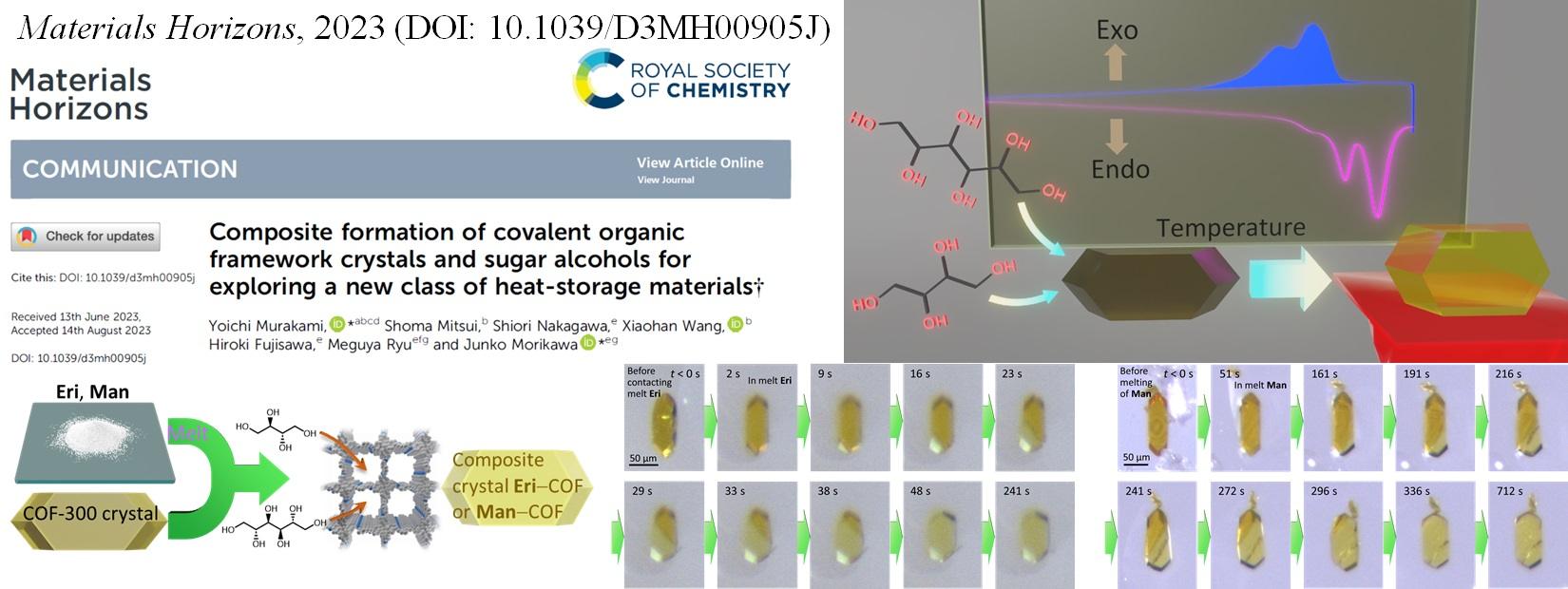
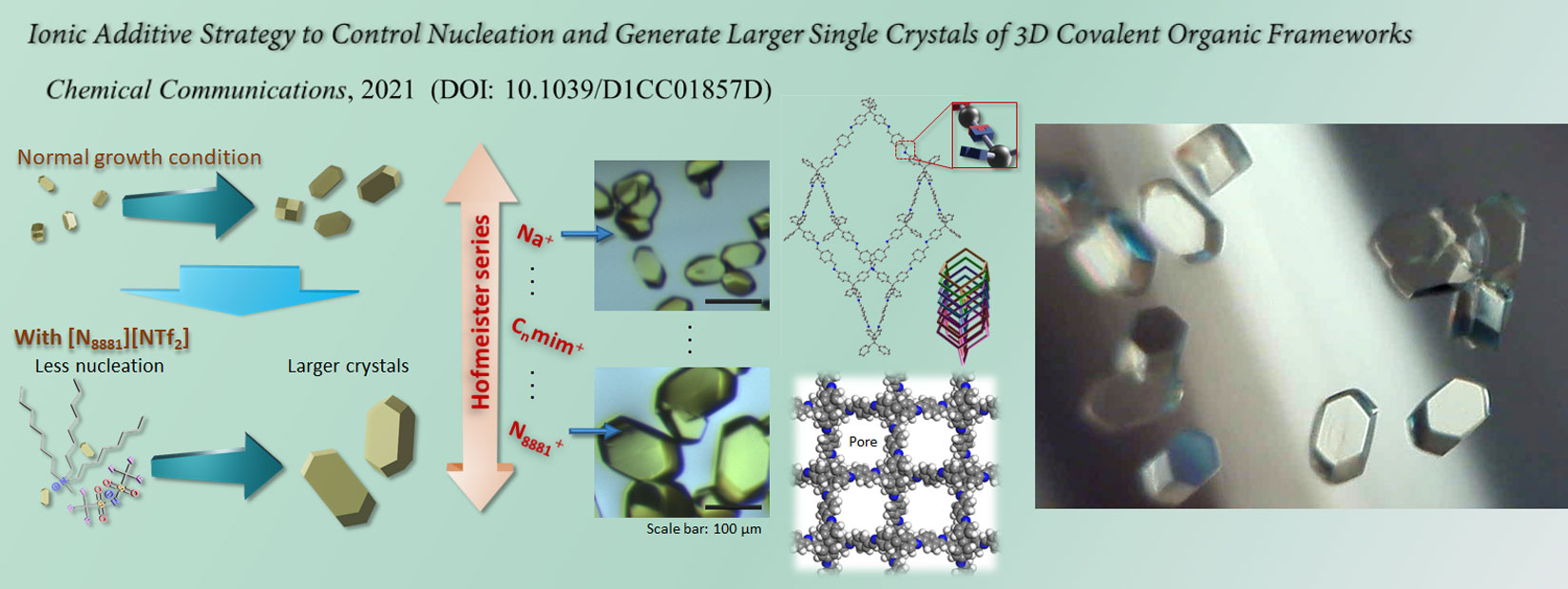
Themoelectrochemical Conversion Integrated into Forced Convection Cooling
In our present civilization, forced convection cooling is important in technologies from microprocessors in data centers, which now consume 1%–2% of global electricity generation, to heat engines including turbines and automobile engines. These technologies require active cooling to avoid thermal failure (for microprocessors) and maximize fuel-to-work conversion efficiency (heat engines). Here, “active cooling” means the prompt removal of a large quantity of thermal energy in the heat source by a working fluid under a large temperature difference. However, such active cooling leads to prompt destruction of the exergy of thermal energy, which is the free-energy component of the thermal energy. This issue has remained unaddressed despite the widespread use of forced convection cooling. If this issue is resolved, it could strongly influence the development of future energy technologies.
The purpose of this research project is to partly recover presently lost exergy in such cooling situations. In particular, we aim at an integration of thermoelectrochemical conversion, which has mostly been studied for stationary conditions using a liquid electrolyte in a closed cell, into forced convection cooling using an electrolyte as the coolant. To fulfill this purpose, we have designed a test cell, in which an electrolyte liquid is forced through a channel formed between two parallel electrodes and the hot-side electrode simulates an object that needs to be cooled.
So far, we have conducted investigations using ionic liquids (room-temperature molten salts composed entirely of ions) as the solvent of redox couple; this solution is forced through the cell as a coolant. The virtual non-flammability and non-volatility of ionic liquids are suitable for wide-ranging heat sources where safety is concerned and situations including uses in space where environment is vacuum, respectively. From our experiments and computational simulations, several findings regarding the fundamental properties of this kind of forced-flow thermocell have been acquired.
However, to bring this concept to real application, further improvements are required. The required improvements include the design optimization of the geometry of liquid flowing channel in the cell, establishment of strategies for scale-up of the cell dimensions, and optimizations of both electrode materials and redox couple chemicals used in the cell.
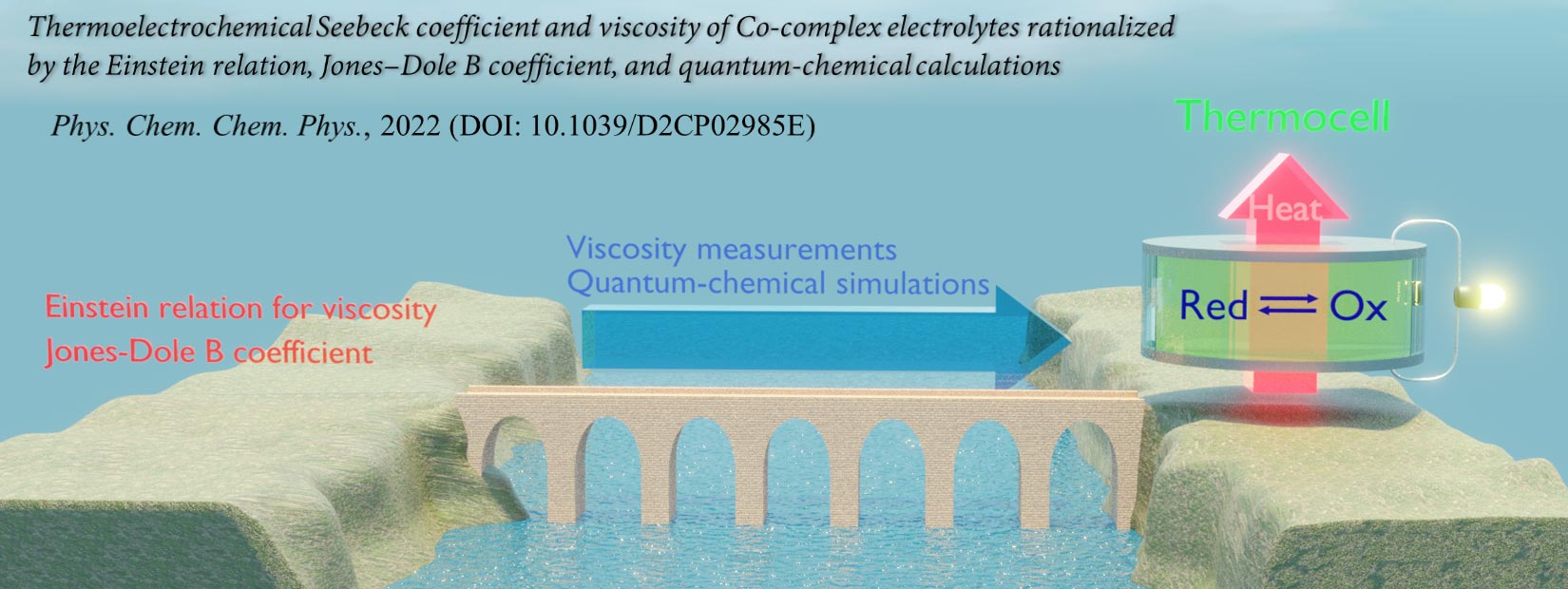
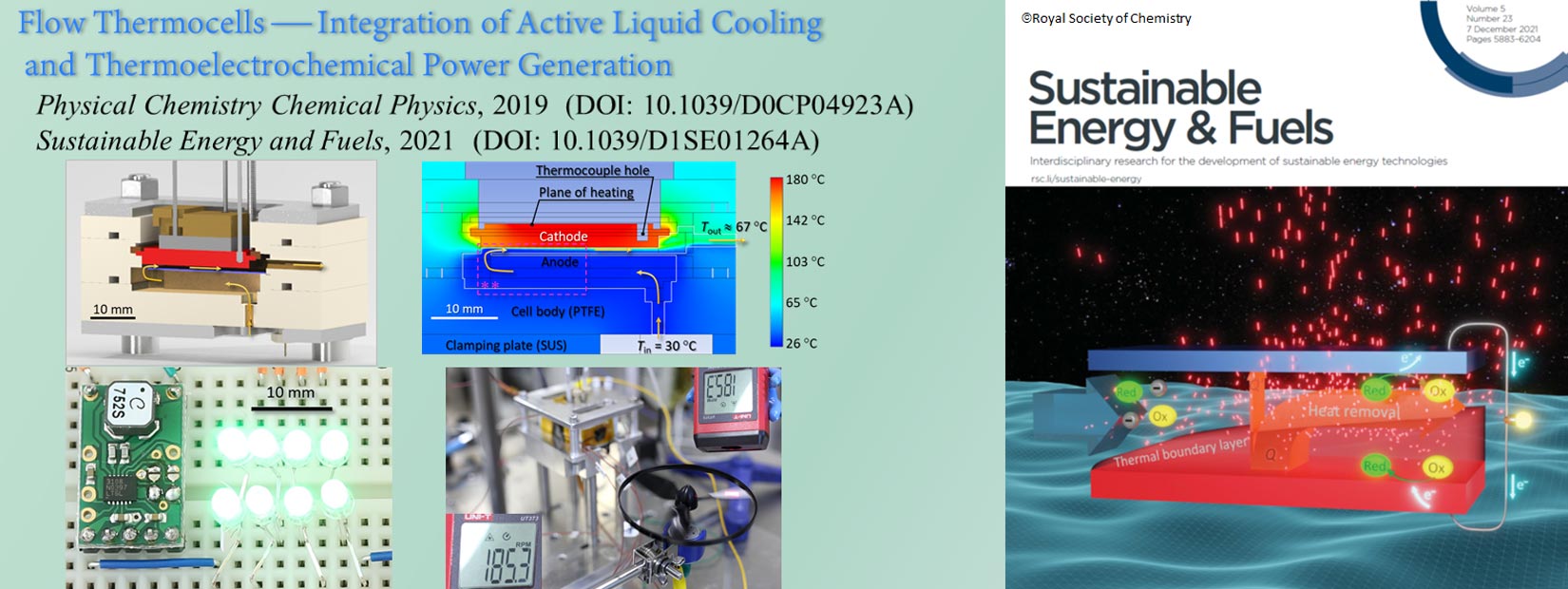
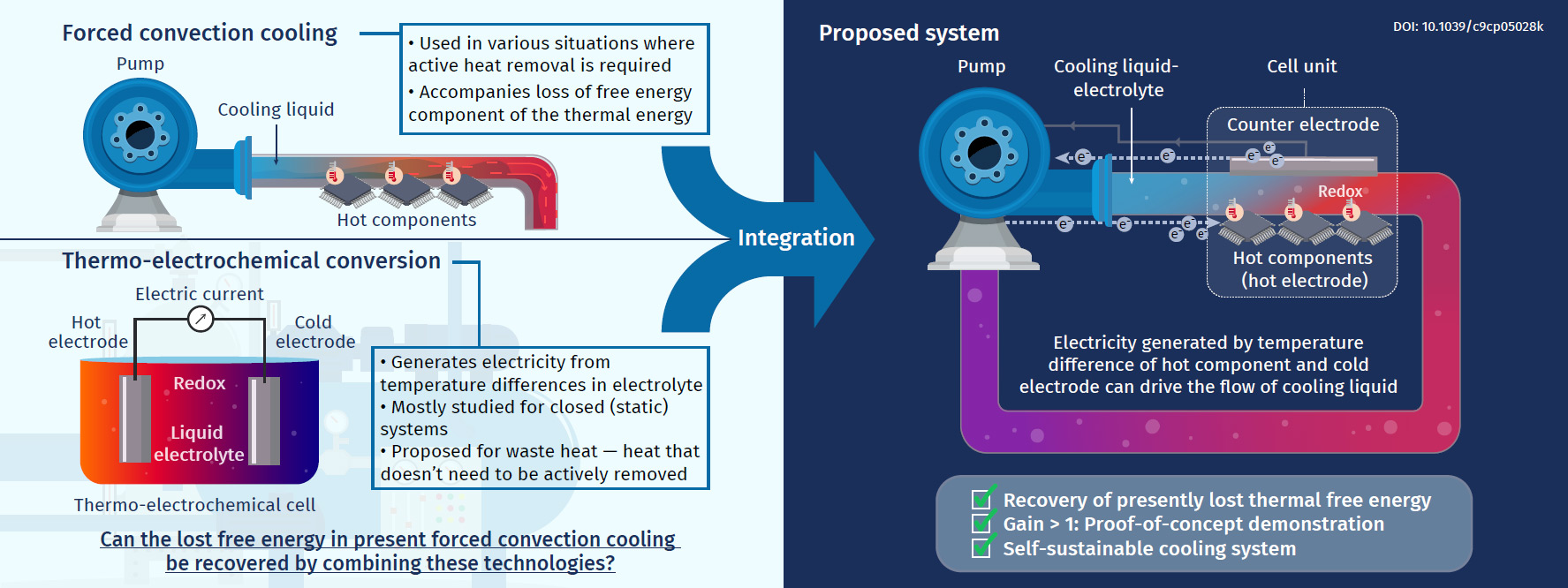
Photon Upconversion using Intermolecular Energy Transfer
Light is an electromagnetic wave, as shown by the fact that monochromatic light obtained using a glass prism has a unique wavelength. Light also behaves as a collection of elementary particles called photons whose existence is manifested by the photoelectric effect. Light energy (photoenergy) is especially important among many possible forms of energy. Sunlight is the only energy supplied to the earth from space. In addition, light has many important practical functions to convert energy and matter to more useful forms. For example, using photovoltaics, photoenergy can be converted to more useful electrical energy. Using photosynthesis materials or photocatalysts, photoenergy can be converted to various storable forms of chemical energy like sugars, proteins, hydrocarbon fuels, and hydrogen gas.
However, the efficiencies of these photoenergy conversion systems suffer from a fundamental limitation. This originates from the fact that only photons possessing higher energies than the bandgap energy of semiconductors or the gap energy between the highest occupied and lowest unoccupied molecular orbitals of molecular materials can be used. Photons with lower energy than these intrinsic gap energies of materials are wasted at present regardless of the power of the incident light. Because the energy of a photon is inversely proportional to the wavelength of light, there is a “threshold wavelength” that represents a boundary separating the usable and unusable spectral regions of sunlight. That is, incident light that has a longer wavelength than this threshold wavelength is useless for photoenergy conversion, resulting in the aforementioned limitation of conversion efficiency. For example, the water-splitting reaction to generate hydrogen gas by a photocatalyst can usually be achieved only by light that has a wavelength shorter than blue (∼450 nm). Photosynthesis in plants can only be driven by light whose wavelength is red or shorter. Light that can generate electric power in amorphous silicon photovoltaics is limited to wavelengths shorter than dark red (∼720 nm). Therefore, given the solar spectrum on earth, numerous photoenergy conversion systems display fundamental losses of energy.
Photon upconversion (UC) is a technology that circumvents these fundamental losses and converts presently unused photons to usable ones. Previously, UC was only possible for high-intensity laser light possessing linear polarization. Recently, a method of UC that uses properly designed intermolecular energy transfer between organic molecules has emerged and found to be applicable to low intensity and randomly polarized light including normal sunlight. Thus, this UC method has great versatility with broader application prospects. Realization of such UC with high efficiency would not only increase the efficiencies of aforementioned photoenergy conversions but also broaden the possible applications of light.
We started studies on this UC technology soon after its emergence and have reported several unique achievements. This research field is currently rapidly growing, through which exciting frontiers and questions are emerging. Our present aims are to elucidate such questions and develop novel UC materials suitable for application. To attain these goals, we are conducting highly interdisciplinary research encompassing physical chemistry, mechanical engineering, photochemistry, and materials science.
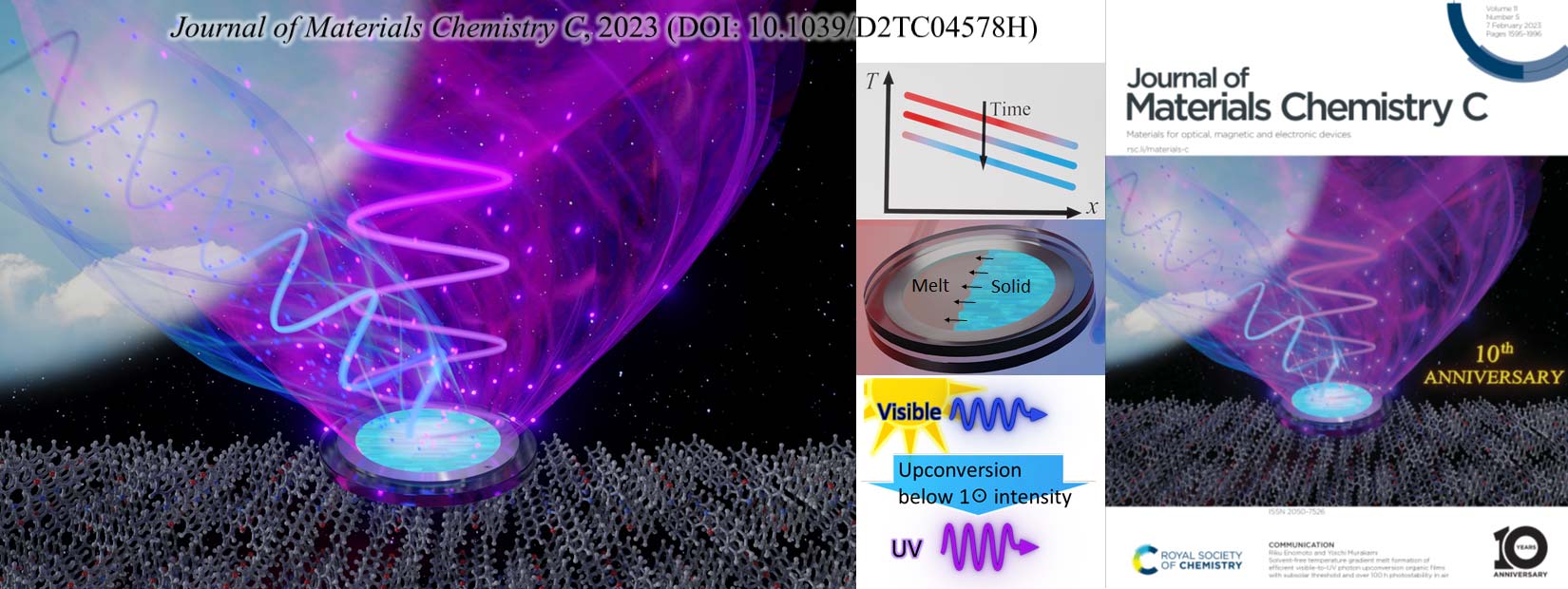
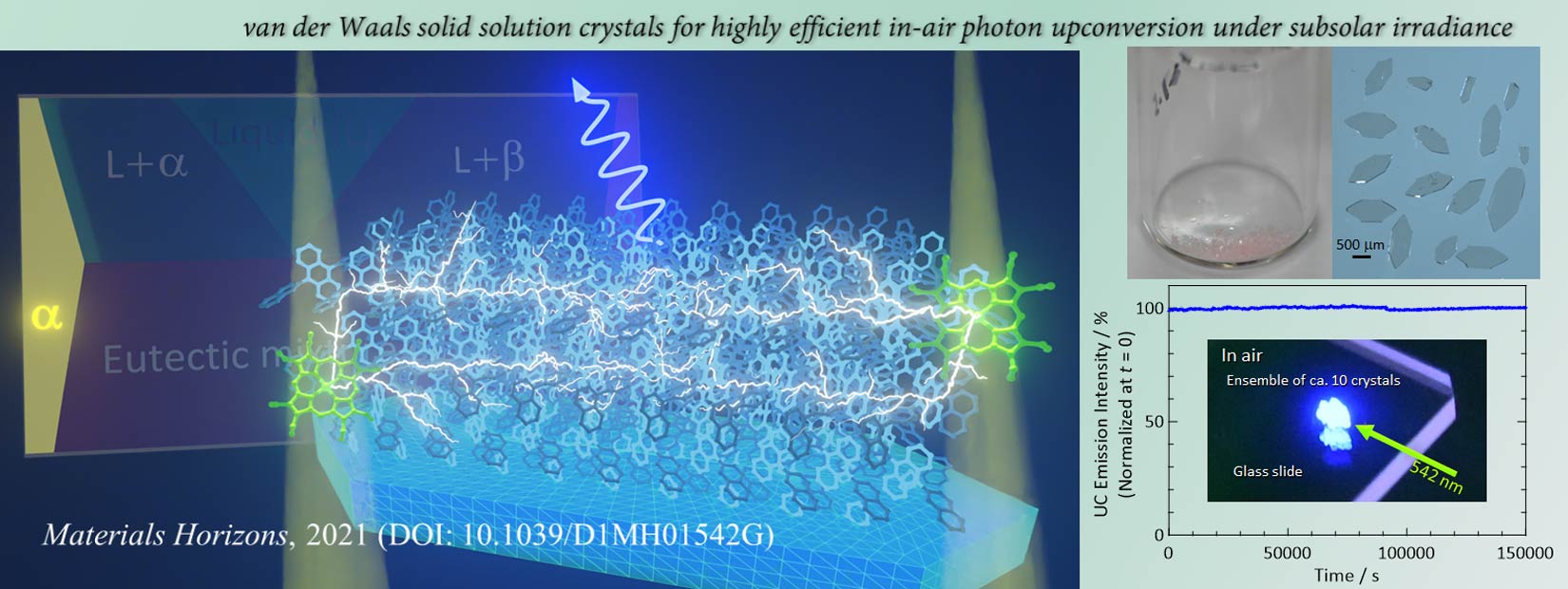
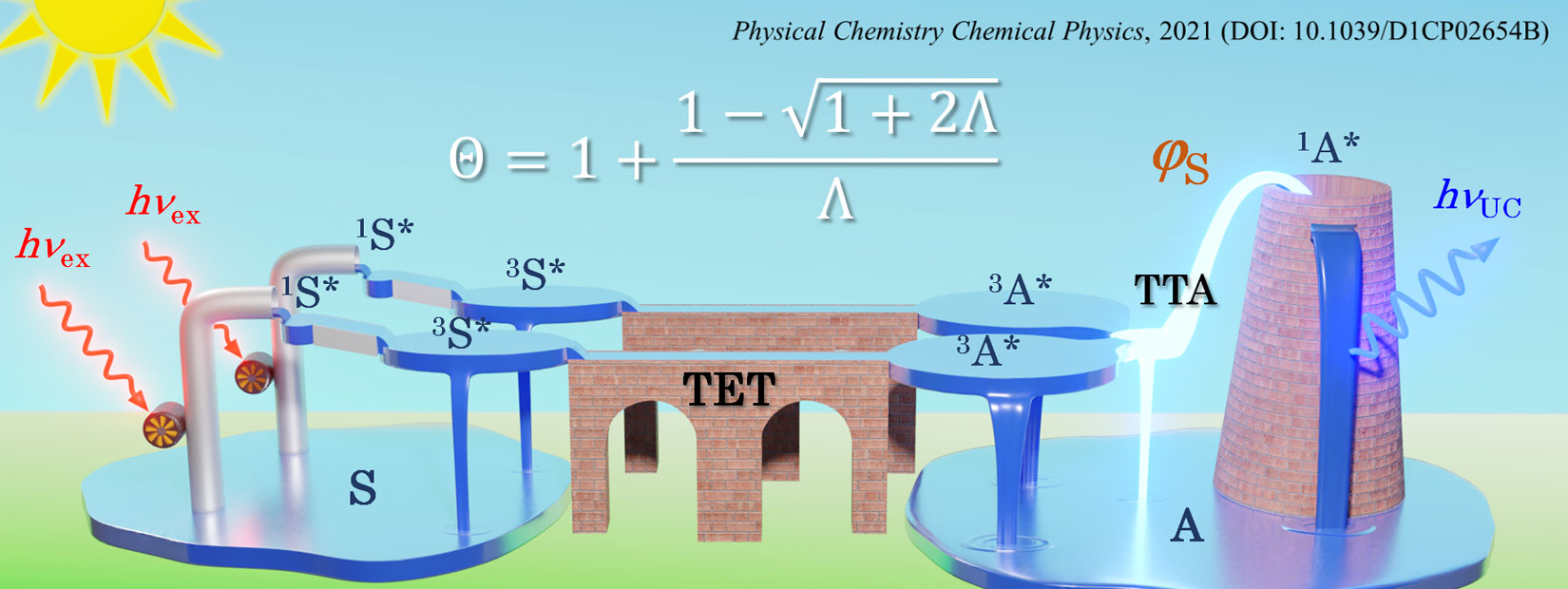